Adaptation to freshwater and hatchery environments in Atlantic salmon
01-14-2021
Writer(s): Avril Harder
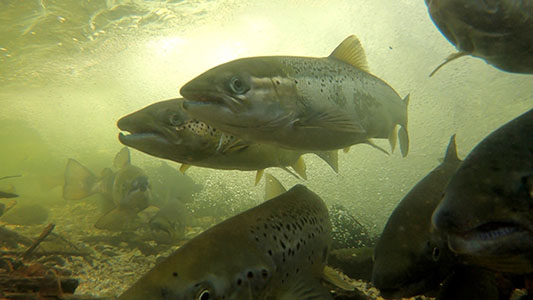
Recipient of the Waser Graduate Assistantship in Ecology & Evolutionary Biology
Atlantic salmon populations are declining throughout their native range, which historically stretched from Long Island Sound, New York to northern Québec in the western Atlantic Ocean. Some of the causes for these declines are, in part, related to salmon life history characteristics. Throughout their life cycle, Atlantic salmon typically migrate between freshwater and marine environments, a strategy referred to as anadromy. Adult salmon spawn and lay their eggs in freshwater streams where their offspring mature before migrating out to the ocean. These offspring continue to grow and mature in the marine environment before migrating back to the stream where they were born to reproduce. Some populations of salmon do occur in freshwater lakes that formed after the North American glaciers receded, and these salmon (often called "landlocked") migrate between the lake environment and streams to complete their life cycles.
For both types of populations, migrations between two different environments make salmon particularly vulnerable to the negative effects of dam construction, which can block migration pathways and decrease the quality and availability of spawning habitat. Indeed, dam construction and historic overharvest of fish in the 1800s initiated the declines in population sizes that continue to this day. In many populations, efforts to maintain or increase population size rely on hatchery support. Eggs are taken from adult salmon as they are returning to spawn and raised in the hatchery until the eggs hatch and the resulting juvenile fish can be released back in to the same population.
Although hatcheries can increase the survival rates of juvenile fish, they can also favor the survival of fish that succeed in captivity but perhaps not in the wild. Hatcheries also usually rely on a small number of adult fish to produce large numbers of offspring, and this can lead to a decrease in genetic diversity in the population. By selecting for fish that do well in captivity and decreasing genetic diversity, hatcheries may actually diminish the ability of a population to respond to future environmental changes.
We used genetic data from three different Atlantic salmon populations (a total of 76 fish) in the U.S. and Canada to explore two main questions:
- How does the hatchery rearing environment affect salmon population genetics?
- How do the two types of Atlantic salmon populations (anadromous and landlocked) differ genetically?
For both of these questions, we also wanted to know which specific genes were involved in potential genetic adaptation.
We compared genetic patterns between two groups of populations that had experienced distinct hatchery rearing environments to identify specific outlier locations in the genome that may be related to hatchery adaptation. Fish from two of the populations (one anadromous, one landlocked) had been reared in the exact same hatchery for two generations prior to sampling, whereas the third population (landlocked) was supported by fish reared in a different hatchery. Previous research has found that genetic adaptation to the hatchery environment, a process sometimes referred to as domestication selection, can occur in as little as one generation. Our results suggest that for the two populations reared in the same hatchery, domestication selection may have already begun to take place after two generations and that fish reared in different hatcheries may be subject to different patterns of selection. We found differentiation between the two sets of populations in genes related to wound healing processes and regulation of circadian rhythm—two processes that have been identified as responding to domestication selection in other species of salmon and trout. Differences between hatcheries with respect to the density at which fish are maintained may lead to selection on wound healing processes due to increased injury rates at higher fish densities, whereas differences in feeding or lighting schedules or even food type may lead to selection on genes involved in circadian rhythm regulation.
Using the same three populations, we also compared two landlocked and one anadromous population to explore patterns of genetic variation that may be related to landlocked populations adapting to an entirely freshwater life cycle. Several genes contained single-nucleotide changes that were strongly differentiated between the population types, including genes involved in immunity. Differentiation in immunity-related genes may be due to the fact that parasite and pathogen communities are quite different between marine and freshwater environments and likely place different demands on the immune systems of anadromous and landlocked fish. We also detected differentiation in genes involved in maintenance of water balance in tissues (osmoregulation), and this pattern is likely a direct result of the founding of landlocked populations by ocean-going fish and subsequent adaptation to an entirely freshwater life cycle. Identification of differentiation in immune- and osmoregulation-related genes is also consistent with previous genetic studies of freshwater populations descended from anadromous populations.
By comparing genome-wide data from three populations, we identified differentiation in genes related to a landlocked life cycle and potentially to the early stages of domestication selection imposed by the hatchery environment. The results of the anadromous vs. landlocked comparison are consistent with previous evidence of local adaptation in salmon and trout populations and highlight the importance of using locally-sourced fish for hatchery supplementation efforts. However, our findings related to domestication selection suggest that adaptation to distinct hatchery environments has occurred over a short timeframe (about 2 generations) in these populations. While hatchery supplementation may help to bolster declining salmon population sizes, these positive demographic effects must be considered alongside the potential negative effects of supplementation on genetic diversity. Loss of genetic diversity that is adaptive in the wild environment may hinder the abilities of hatchery-supported populations to respond to future environmental change.